Regenerative medicine could pave the way to treating baldness
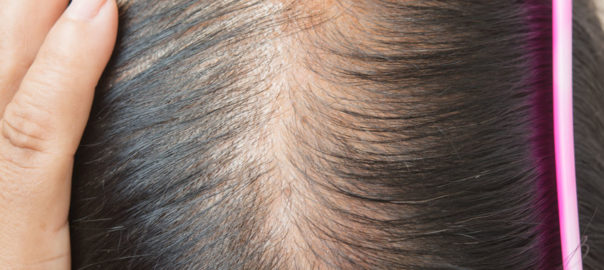
When hair follicles were first generated from stem cells that had been isolated from adult mouse skin1, Jay Leno — a former host of US talk show The Tonight Show — joked that scientists “cured baldness … at least in mice”. Sixteen years on, the current host will have the opportunity to mention that scientists have ‘cured’ baldness in humans, now that Lee et al.2, writing in Nature, have regenerated hair follicles from human stem cells. This achievement places us closer to generating a limitless supply of hair follicles that can be transplanted to the scalps of people who have thinning or no hair. Moreover, if the approach reaches the clinic, individuals who have wounds, scars and genetic skin diseases will have access to revolutionary treatments.Read the paper: Hair-bearing human skin generated entirely from pluripotent stem cells
Research into skin-tissue engineering began in 1975, when a landmark study showed that cells called keratinocytes could be isolated from the surface layer of a person’s skin (the epidermis)3, and the cell population expanded in culture. Almost a decade later, sheets of keratinocytes isolated from people with burns were transplanted back to the individuals they came from as life-saving, permanent engraftments4. This work was the foundation for another remarkable achievement in 2017, when a boy who had a genetic disease called junctional epidermolysis bullosa (which causes severe fragility of the skin) had his epidermis replaced with genetically corrected cells5. For this type of cell-based approach to advance further, grafted skin must include more of the components found in normal skin: hair follicles, pigment-producing melanocyte cells, sweat glands, nerves, muscle, fat and immune cells, in addition to epidermal cells.
Enter Lee and colleagues. The authors leveraged research from the fields of stem-cell biology and hair-follicle development6 to generate near-complete skin organoids — self-organizing tissues grown in the laboratory that mimic developing skin. Organoids have been grown to imitate various organs, including the gut, lung, kidney and brain7. Because organs consist of many cell types, organoids are typically formed from pluripotent stem cells, which have the ability to form all adult cell types. These can be embryonic stem cells or induced pluripotent stem cells, which are created by reprogramming adult cells to an embryonic-like state8.
The epidermis and the dermis — the skin’s other main component — are derived from different cell types in the early embryo. Lee et al. optimized the culture conditions needed to generate skin organoids containing both components from human pluripotent stem cells. The authors sequentially added growth factors to the stem cells. First, they used BMP4 and an inhibitor of the transcription factor TGF-β to induce formation of the epidermis. Next, they treated the cells with the growth factor FGF2 and an inhibitor of BMP, to induce the formation of cranial neural crest cells, which give rise to the dermis.
The cells grew in a sphere. After more than 70 days, follicles began to appear, which ultimately produced hair (Fig. 1). Most hairs were pigmented by melanocytes, which also develop from the cranial neural crest. Tissues associated with hair follicles — such as sebaceous glands, nerves and their receptors, muscles and fat — developed, too, leading to the formation of remarkably complete skin in a dish9. One missing component, however, was immune cells, which normally reside in and around hair follicles, and have diverse roles in the skin10.
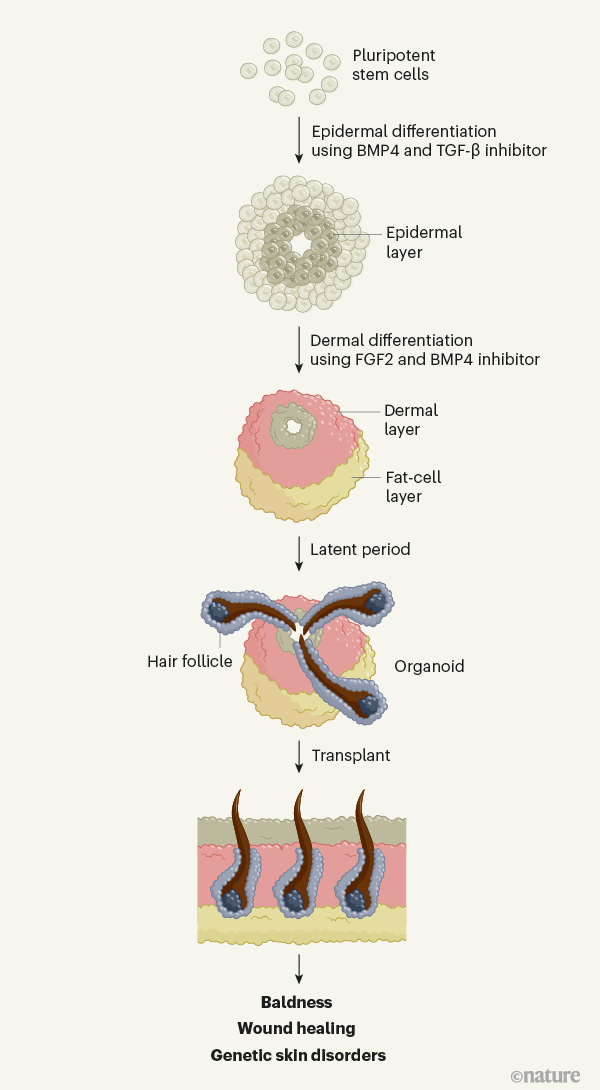
Lee and colleagues found that their organoids expressed genes that were characteristic of skin from the chin, cheek and ear. Interestingly, dermal cells on the scalp might also derive from the neural crest11. This suggests that the organoids might actually mimic scalp skin, and also highlights that it could be possible to tailor the authors’ protocol to generate skin that has the characteristics of different body sites, by altering the culture conditions in which the cells are grown.
The group’s organoids will be a perfect tool for analysing the roles of various biological pathways in skin development — small-molecule inhibitors or inhibitory RNA molecules can be used to block proteins or pathways and to investigate the effects on skin growth. The organoids can be used in combination with genome-wide association studies or other genetic data to analyse how particular genetic mutations alter skin development. They can also help to model diseases of the skin and hair and to screen experimental drugs for any toxicities and for their efficacy.How the stress of fight or flight turns hair white
Beyond these in vitro benefits, the authors demonstrated that the organoids have therapeutic potential in vivo. They transplanted the organoids onto immunodeficient mice (to ensure the graft was not rejected by the animals’ immune system), and showed that just over half of organoids go on to form hair, which is distributed over the surface of the graft. This illustrates the exciting potential of introducing skin organoids into wounds to encourage healing and prevent scarring, or transplanting them into areas lacking hair.
However, several questions remain before this therapeutic approach becomes a reality. For instance, how efficiently and reproducibly do hairs develop? How many cells are needed to eventually form a hair follicle once grafted? Lee et al. began to answer the first of these questions by showing that a separate laboratory could grow hair in organoids using the same culture conditions. However, dealing with variability between individual stem cells and between the stem cells from different people are daunting challenges.Advances in mini-brain technology
The prolonged length of time required for organoids to develop hair follicles mimics fetal skin development12. Similarly, in both settings, the skin undergoes a latent ‘resting’ phase before follicles begin to grow. This is a fascinating area for future study. However, it took 140 days before organoids were ready for engraftment, which could impede the therapeutic potential of the work — someone with burns, for instance, cannot wait that long for a skin graft. Further studies to understand the molecular events taking place during this latent phase should provide strategies for accelerating this process using molecules that alter relevant signalling pathways.
Several other aspects of the authors’ approach will also need to be optimized before it can move to the clinic. The hairs that grew in the current study were small; in future, further optimization of culture conditions will be needed to form large scalp hairs. Better characterization of some components used in the culture cocktail — such as a protein mixture called Matrigel — will be necessary to ensure that they comply with good manufacturing practices. And future work might need to move away from using pluripotent stem cells, which can have undesirable side effects, such as promoting tumour formation. An appealing alternative might be to use adult stem cells.
Despite these caveats, Lee and colleagues’ study is a major step towards a ‘cure’ for baldness in humans, and paves a way towards other, greater therapeutic possibilities. At a minimum, it is worth a shout-out on a late night show. The work holds great promise of clinical translation — we are confident that research will eventually see this promise realized.
Credit: Nature.com Leo L. Wang & George Cotsarelis https://www.nature.com/articles/d41586-020-01568-2